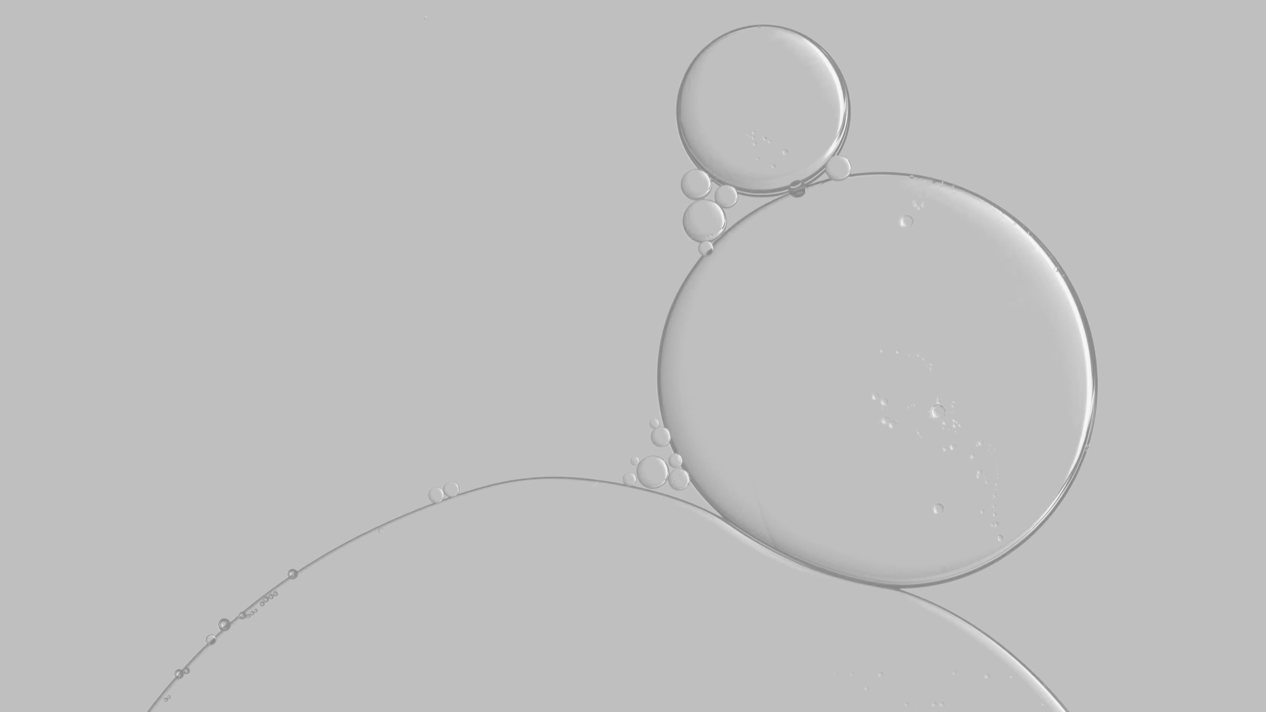
OBESITY
The prevalence of obesity has increased and continues to increase at an alarming rate in our society, as well as in countries with economies in transition, taking on epidemic proportions. In Spain, the most recent data on prevalence in adults, obtained from the ENRICA study in 2011, provide a prevalence of obesity of 22.9% and overweight of 39.4% over a population of 12036 people surveyed. Epidemiological data in Spanish children (ALADINO study) show a high prevalence, with 45.2% being overweight, of which 26.1% are overweight and 19.1% obese. The kilos gained by Spaniards and Europeans have disastrous consequences for public health and represent an enormous economic expense calculated at between 2 and 8 percent of health expenditure. The most serious consequences for health include the development of associated diseases such as type 2 diabetes, cardiovascular diseases, certain types of cancer, as well as psychosocial problems.
Although obesity can be explained by genetic alterations, the current epidemic is mainly caused by external factors such as modern lifestyle, diet and, above all, the absence of physical activity; thus, obesity can be defined as a multifactorial pathology that requires long-term treatment including diet and exercise. So far the number of drugs that have been developed for the treatment of obesity is very limited and not very successful. For this reason, the development of new and better anti-obesity treatments, which together with diet and exercise contribute to a reduction in body weight, requires a better understanding of the mechanisms at a molecular level that control the energy balance.
ENERGY BALANCE. The control of intake and body weight is characterized by a complex interaction between peripheral organs involved in energy homeostasis such as adipose and muscular tissue, thyroid, liver and gastrointestinal tract, and the central nervous system (CNS). The peripheral tissues inform the hypothalamic appetite regulating centers about the nutritional and metabolic status by releasing various circulating signals such as glucose, insulin, leptin and ghrelin among others (Berthoud and Morrison, 2008). How adipose tissue functions as an energy store in the form of triglycerides, is the major controller of fatty acid release playing a critical role in the development of insulin resistance in many pathological situations. Many studies have shown that excess adipose tissue or ectopic lipid accumulation is responsible for the metabolic disorders that cause type II diabetes and dyslipemia. Adipose tissue releases a wide range of hormones of peptide nature or adipokines involved in the regulation of energy metabolism, and is also a major producer of inflammatory cytokines related to the deregulation of metabolism in obesity. Adipokines may play a key role in linking fat tissue accumulation and insulin resistance. Among the already known adipokines, e.g. leptin, adiponectin, retinol-binding protein-4, visfatin, adipsin, TNF-1 and interleukins 6, 11, very few have been defined as regulators of insulin sensitivity, metabolism and energy homeostasis. In this context, the discovery and characterization of new factors derived from adipose tissue is still in progress.
Fetuin-A: Fetuin-A, also called (alpha2-Heremans-Schmid glycoprotein, AHSG) is an abundant serum protein (Dziegielewska et al., 1990) so far exclusively produced by the liver, tongue, and placenta (Denecke et al., 2003). In several studies, fetuin-A has been shown to act as a natural insulin tyrosine kinase receptor inhibitor in both the liver and skeletal muscle (Mathews et al., 2000). Mice deficient in the fetuin-A coding gene showed increased sensitivity to insulin and were resistant to diet-induced weight gain (Mathews et al., 2002). In addition to the effects of fetuin-A on the insulin receptor in liver and muscle, it is postulated that fetuin-A may be involved in another mechanism through which it affects adipose tissue to induce widespread insulin resistance throughout the body. Polymorphisms of the fetuin-A gene have been shown to be associated with type 2 diabetes (Siddiq et al., 2005) and affect the action of insulin on adipocytes (Dahlman et al., 2004). In addition, fetuin-A has been shown to have direct pro-adipogenic properties (Schmidt et al., 1990), however the mechanisms involved in this process are unknown. More recently it has been shown that levels of this protein in human plasma correlate with fatty liver, glucose intolerance, insulin resistance, metabolic syndrome and atherogenic lipid profile (Stefan et al., 2006; Mori et al., 2006; Ix et al., 2006). In addition, Hennige and colleagues have found that fetuin-A induces low-grade inflammation and suppresses adiponectin production in animals and humans (Hennige et al., 2008).
Multiple studies have shown the relationship between obesity and inflammation, but recent articles point to fetuin-A as a mediating protein between excess lipids and activation of the innate immune system in adipose tissue, which ultimately results in the release of cytokines and inactivation of the insulin receptor (Pal et al., 2012; Bucci et al., 2013). It has been observed that fetuin-A acts by presenting free fatty acids to the Toll 4 receptor (TLR4). This receptor is found in macrophage and adipocyte membranes, and its physiological function is to recognize molecular patterns of pathogens and trigger the immune response to fight them. However, it has been shown that TLR4 can also be activated in the absence of microorganisms by interaction with free fatty acids, when these are in excess and bound to fetuin-A. Activated TLR4 causes the activation of the nuclear transcription factor NF-КB, which results in the expression of pro-inflammatory cytokines. The constant release of these cytokines eventually leads to insulin resistance by the receptor, which leads to glucose intolerance (Pal et al., 2012). Another subsequent study has revealed an alternative mechanism by which fetuin-A mediates the activation of adipose tissue inflammation in response to excess fatty acids at the paracrine level. It has been shown that excess lipids in the adipose tissue environment lead to increased expression of fetuin-A by adipocytes. Fetuin-A is secreted to the extracellular medium and acts, in synergy with other molecules, as a chemotherapeutic agent by attracting M2-type macrophages to the adipose tissue (Chatterjee et al., 2013). Furthermore, it has been observed that fetuin-A induces the polarization of these M2 macrophages (with anti-inflammatory phenotype) towards M1 type, which release pro-inflammatory cytokines. The authors propose that the release of these molecules into the adipose tissue has a paracrine effect by inhibiting the insulin receptor present in the adipocytes themselves (Chatterjee et al., 2013). Thus, it is shown that fetuin-A exerts its insulin receptor inhibitory action through at least three different mechanisms: direct interaction, activation of the TLR4 receptor, and attraction and polarization of macrophages (Goustin et al., 2011; Pal et al., 2012; Chatterjee et al., 2013).
MUSCLE TISSUE AS AN ENDOCRINE ORGAN:
In addition to the classic endocrine organs, several tissues such as fat tissue have been found to have physiologically relevant secretory activity. The potential role of skeletal muscle tissue as an endocrine organ was first suggested when the expression and secretion of interleukin-6 (IL-6) in response to muscle contraction was discovered (Febbraio and Pedersen 2002). In this context, Pedersen and collaborators established a new paradigm by pointing out that muscle tissue is capable of secreting hormone-like factors called myokines that could affect the metabolism of other tissues and organs (Pedersen and Febbraio 2008; Pedersen 2011). Proteins secreted by skeletal muscle may act locally in muscle cells in an autocrine/paracrine manner, on nearby tissues such as muscle blood vessels, or may be released into the circulation producing effects at the systemic level. In particular, the myocines secreted during muscle contraction may exert a protective role against diseases associated with sedentary lifestyles (Pedersen 2009).
FNDC5/IRISIN. In January 2012, Spiegelman's group and collaborators published in Nature the discovery of a new myochine secreted by the muscle they called irisin (NDC5/irisin) (Bostrom et al., 2012). In this work, they describe that irisin secretion is induced by physical exercise and depends on the transcriptional co-activator PGC1alpha. The most relevant aspect of the work is that it seems to be able to bind to as yet unknown receptors of white adipose tissue precursor cells inducing their differentiation into brown adipose tissue (TAP) in a process called "browning". For this purpose, irisin would induce the expression of UCP-1 and other genes associated with TAP through the expression of the nutritional sensor PPARgamma. The relevance of this finding, if confirmed, is promising as a new tool for the treatment of obesity and diseases associated with sedentarism since browning is specialized in dissipating energy in the form of heat. Irisine-induced differentiation of white to brown adipose tissue would improve the metabolic profile of this tissue and increase the overall energy expenditure of the whole body.
The discovery of FNDC5/irisin has caused a great stir in the scientific community. However, at this time there is some controversy surrounding the newly discovered mycokine irisin and its secretion in humans after exercise as well as its true relationship to metabolic status (Timmons et al., 2012; Moreno-Navarrete et al., 2013). Very recent studies, among them from our group, show that contrary to expectations, animals and obese patients present elevated circulating levels of this protein compared to healthy individuals (Huh, Panagiotou et al. 2012; Stengel, Hofmann et al. 2012) suggesting a resistance to this hormone in these cases of increased body mass index (BMI) (Roca-Rivada., et al. 2013; Crujeiras et al., 2014; Pardo et al., 2014). Thus, after the publication of the first paper describing the existence of this myochin, several authors questioned the true nature of the secreted form of CDF5. Our group, which has specialized in recent years in the characterization of new signals released by adipose and muscle tissue (Roca-Rivada et al., 2011; Roca-Rivada et al., 2012), has recently pioneered the study of direct irisin secretion from explants of muscle tissue in vitro (Roca-Rivada, et al. 2013). Our work has provided new information on the mode of irisin secretion by showing that skeletal muscle tissue is capable of secreting a form similar to that described in Nature of 25kDa using antibodies against both the intracellular and extracellular part of the protein. These results suggest that the secreted form may correspond to the complete protein. In this work we also described differences in irisin secretion depending on the muscle type; thus we showed for the first time that muscle tissue composed of oxidative-slow type fibers secretes 40% more irisin than that formed by glycolytic-fast fibers. Taking into account that physical training increases oxidative fibres, our results would confirm the link between exercise and irisin secretion. Thus, in both muscle types we found that the secretion of this myochin increases after 3 weeks of exercise as described by Spiegelman in vivo with rodents (Bostrom, Wu et al. 2012).
FNDC5/IRISIN IS NOT ONLY A MYCOKINE, BUT ALSO AN ADIPOKINE:
Under the previous context, during the characterization of irisin secretion from muscle tissue, our group has established a new paradigm in relation to FNDC5/irisin by discovering that this myochin is also expressed and secreted by white adipocytes from adipose tissue in rats and humans, and therefore can behave like an adipokin (Roca-Rivada et al., 2013). Specifically, our first results with direct secretion studies from rat explants show that visceral and especially subcutaneous adipose tissue express and secrete CDF5. In comparison with muscle tissue, we found that subcutaneous adipose tissue secretes 20% and visceral tissue 40% less iridescence than the more secretive oxidative type muscle under normal conditions. We have also observed that adipose tissue increases the secretion of FNDC5/irisin after periods of moderate physical exercise, but shorter than in muscle, while fasting decreases it. Consequently, the adipose tissue of anorexic animals hardly showed secretion of this protein, while that from obese animals secreted significantly more FNDC5/irisin than the control animals. These results were also confirmed at the circulating level showing that contrary to expectations, obese animals showed very high levels of this protein. Thus, we postulated for the first time that adipose tissue could contribute to circulating FNDC5/irisin, and that this contribution would vary depending on the physiological status or pathological situation (Roca-Rivada., et al. 2013). We suggest that under normal conditions it would be the muscle that would actively participate in the circulating irisin levels, while in pathological situations with high adiposity such as obesity, it would be the adipose tissue that would be responsible. Preliminary studies by our group analyzing circulating levels in obese individuals are confirming our hypothesis by positively correlating irisin levels with adiposity factors such as BMI, waist diameter, and kg of fat mass (Crujeiras et al., 2014; Pardo et al., 2014). In addition, it is important to highlight the recent work of Stengel and collaborators showing high circulating levels in obese patients compared to normopose and anorexic patients, and the also positive correlation between irisin and weight, and fat mass (Stengel et al., 2013). Given the beneficial effects attributed to irisin, it could be suggested that exposure to high levels of this protein in obese patients may be responsible for resistance to it. Supporting this hypothesis are studies that show that obese subjects possess very low percentages of brownish adipose tissue compared to normopes (Virtanen et al., 2009).
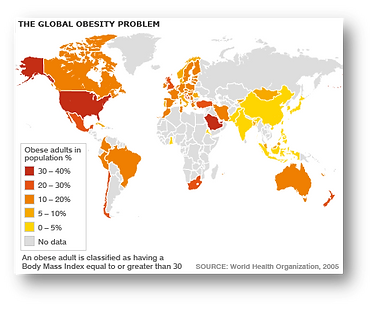
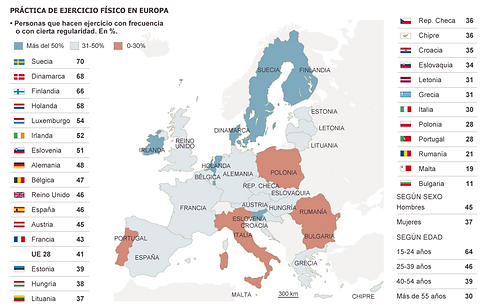
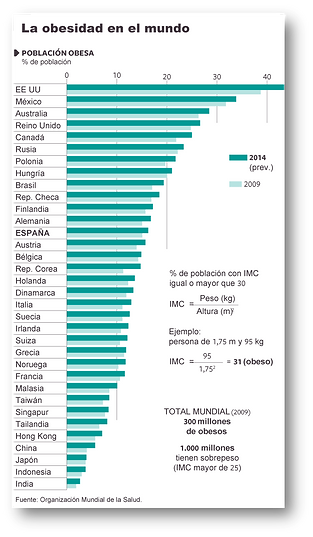
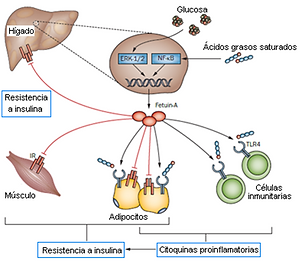
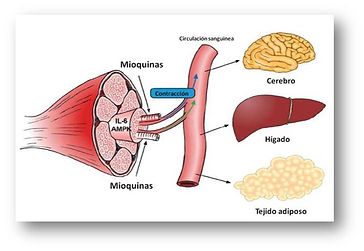
